ASSESSMENTS
Approaching a Quantum Leap in Computing
Jul 24, 2015 | 09:01 GMT
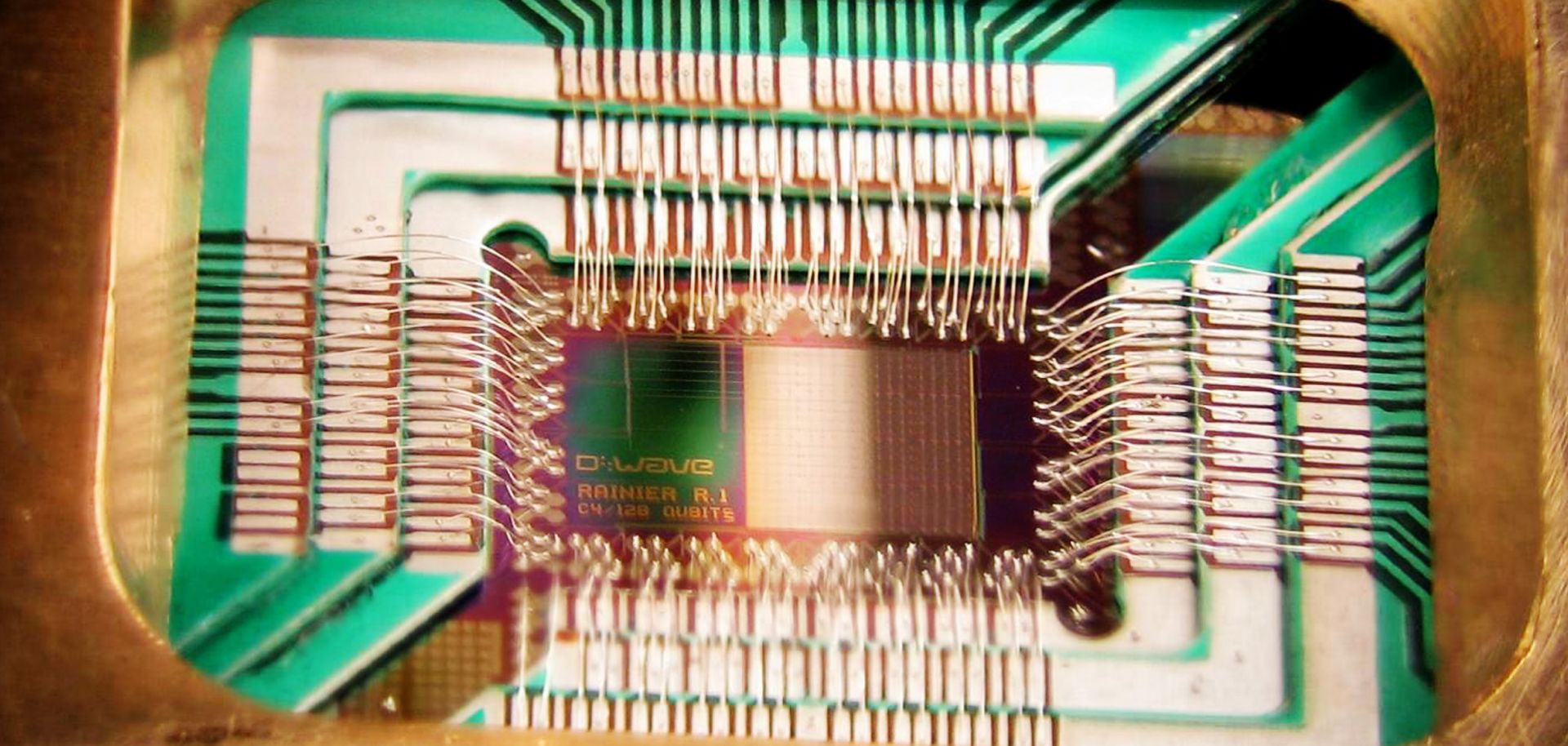
A D-Wave Systems chip designed to operate as a 128-qubit superconducting adiabatic quantum optimization processor.
(D-Wave Systems, Inc.)
Forecast Bullets
- The widespread use of quantum computers in industry is likely only a decade or two away.
- The United States will probably maintain its lead in the field, though China will be competitive.
- The countries and companies that first access quantum computers will enjoy a powerful advantage over their peers in areas that stand to gain from the technology.
Subscribe Now
SubscribeAlready have an account?